Defining the level of indoor comfort for a building in
accordance with the climatic conditions found on site, has enormous potential
for saving energy at absolutely no cost. Adjusting the thermostat in hot climates from 24°C to
28°C results in energy savings of 50 to 65 %, while maintaining good thermal
comfort for the occupants. In cooler climates, reducing the thermostat
temperature by 5-8°C for 8 hours a day (e.g., at night), will result in energy
savings of up to 15%.
Introduction
Humans can tolerate a wide range of climatic conditions. However, they prefer a condition where the optimum conditions of thermal comfort are maintained. The thermal comfort zone has been defined as the condition under which man succeeds in arriving at “the point at which minimum expenditure of energy is needed to adjust himself to his environment" (Olgay, 1963). It is thus a description of the thermal relationship between man and his immediate surroundings.
Heat exchanges of the human body
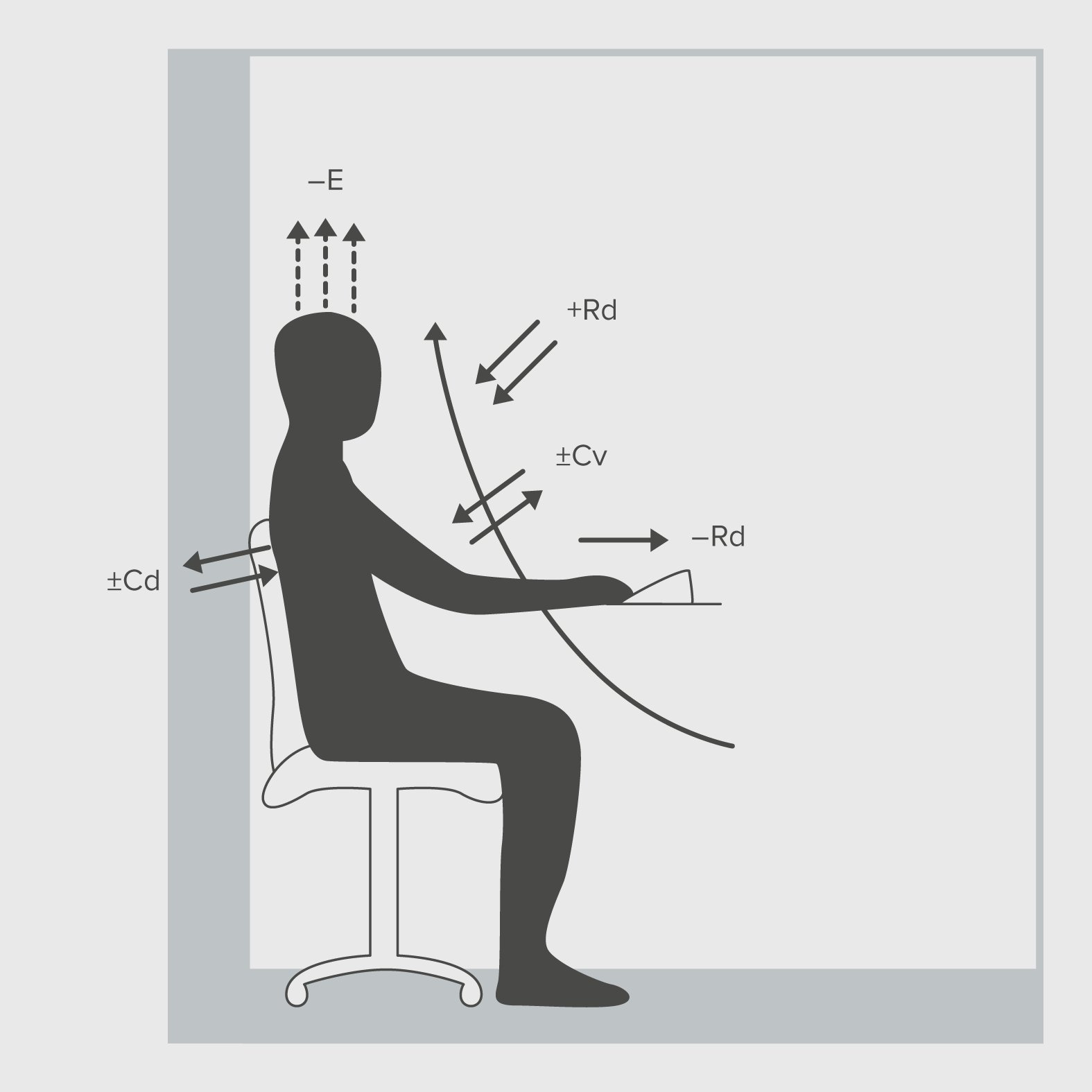
(Szokolay, 2008)
Cd = conduction
E = evaporation
Rd = net radiation exchange
Cv = convection (including respiration)
Thermally comfortable environment is one of the most important factors in building design (Griffiths, 1988). It defines among other factors the indoor climate conditions of buildings and thus the conditions set through heating, cooling and dehumidification and the energy consumption of the technologies. Conditions outside of the thermal comfort zone can be described as thermal stress which maybe caused by a single factor or a combination of factors. Thermal stress can cause among other things headaches, drowsiness and in extreme cases heat stroke.
Although a definite description of thermal comfort is extremely hard as it is based on the personal per-ception of the thermal comfort. It can be defined through a number of factors which effect the level of comfort provided. Thermal comfort is defined by up 21 factors. Studies have shown however that only 6 of these are primarily dominant. These factors being:
Environmental Factors
- Air temperature
- Radiation
- Air velocity
- Humidity
Personal Factors
- Clothing
- Activity Level (Metabolic rate)
Other (less dominant) factors
- Gender
- Health
- Nutrition
- Age
- Acclimatization
- As well as psychological factors such as expectation
Overview
Taking the ability to adapt to an environment into consideration, building occupants in hot climates, do find indoor temperatures as high as 32 °C acceptable during the hottest days of the year, with light clothing and control over the immediate environment like windows and fans. Acceptable temperatures are of course different from preferred temperatures; at an indoor temperature of 32 °C, a majority of occupants may very well prefer a cooler temperature. The potential of the adaptive approach is enormous, especially because these energy savings can be achieved at absolutely no cost. The thermal ‘shock’, often experienced when moving from air-conditioned spaces to the outdoors and vice versa, is also drastically reduced when using this adaptive approach and leads to an altogether improved thermal comfort. In addition, increasing the air speed through the spaces can significantly increase thermal comfort conditions for the occupants through a cooling sensation on the skin. To maintain comfort conditions, the air speed should increase from 0.4 m/s at a temperature of 24-27 °C to above 0.8 m/s at temperatures as high as 29-32 °C.
In addition to temperature and air speed considerations, aspects of relative humidity must be considered as well. In hot and arid regions, it is important to maintain relative humidity levels above 35 % for comfort. In hot and humid regions, humidity levels should not rise beyond 80% to maintain comfort conditions.
Human behaviour
In actively conditioned buildings a thermostat setting of 28 °C can yield substantial energy savings of 50 to 65 % (i.e. reducing energy consumption by a factor of 2-3) compared to the common practice of cooling buildings to 23-24 °C (Harvey, 2006). Increasing the thermostat setting from 23 °C to 27 °C for night-time air conditioning of bedrooms in apartments in Hong Kong, also results in energy savings of a factor of 2-3 (Lin and Deng, 2004).
Example for Mumbai
Acceptable indoor temperatures during the hottest month
(Average outdoor temperature of 30 °C in May)
Upper thermal comfort limit acceptable by 80%/90% of occupants: 30.6 °C/29.6 °C
Acceptable indoor temperatures during the coolest month
(Average outdoor temperature 24.2 °C in January)
Upper thermal comfort limit acceptable by 80%/90% of occupants: 28.8 °C/27.8 °C
The thermal comfort limits for hot and arid climates e.g. Jodhpur – India, should be defined as 23.3 °C (lower limit) and 28.3 °C (upper limit).
In cool and temperate climates, much lower temperatures are acceptable in winter with warm clothes and control over the immediate environment like radiators and windows. The minimum temperature acceptable in a building on very cold days is as low as 16 °C. Acceptable temperatures are of course different from preferred temperatures. Again, most occupants would most likely prefer higher indoor temperatures. However, by adjusting the thermostat from 22 °C to 20 °C, savings in heating energy in the range of 12% can be achieved.
In winter, heating energy can also be reduced by setting the thermostat to 20°C -whilst awake - and then setting it lower during the night or when away from home. By turning the thermostat down to 12-15 °C for 8 hours, additional savings of about 5%–15% a year can be achieved - savings of as much as 1% for each degree are achievable IF the ‘setdown/setback’ period is eight hours long. The percentage of savings from ‘setdown/setback’ is greater for buildings in milder climates than for those in more severe climates.
Air movement should also be kept to a minimum in order to minimize body heat loss by providing only the fresh air needed to maintain healthy air quality. In summer, air movement should be maximized when cooling is needed through the use of individually controlled ceiling and/or desk fans.
Example for Quebec
Acceptable indoor temperatures during the warmest month
(Average outdoor temperature of 20.7 °C in July)
Upper thermal comfort limit acceptable by 80%/90% of occupants: 27 °C / 28 °C
Lower thermal comfort limit acceptable by 80%/90% of occupants: 21 °C / 22 °C
Acceptable indoor temperatures during the coolest month
(Average outdoor temperature -10.8 °C in January)
Upper thermal comfort limit acceptable by 80%/90% of occupants: 22 °C / 23 °C
Lower thermal comfort limit acceptable by 80%/90% of occupants: 15.7 °C/16.7 °C
Technique
These can be divided into two types, the Heat Balance Approach and the Adaptive Thermal Approach. The most widely used and internationally accepted are the ISO 7730 (2005) which is a heat balance approach and the ASHRAE 55 (2004) and CEN 15251 (2011) which are adaptive approaches. Although each model can be used in all conditions norms such as the EN 15251 recommend that the heat bal-ance approach (Fanger Model) be used in actively controlled buildings whereas the adaptive approach is used in open buildings and non-mechanically ventilated buildings.
The most widely used international standard for thermal comfort, developed by Povle Ole Fanger, based on predicted using mean vote (PMV) and percentage of dissatisfied people (PPD) and is used in ISO 7730 (Fanger, 1972). Empirical equations to determine PMV and PPD have been developed by surveys conducted in closed and thermally controlled chambers based on a seven point (-3 to +3) scale ranging from too hot to too cold. The thermal comfort range can be determined through PMV. From this PPD can be calculated. It is customary to define thermal comfort by a ‘comfortable’ or the ‘comfort zone’, in which 80% of all occupants are satisfied with their environment (PPD 20%). That means they feel neither too cold nor too hot. A slightly stricter definition of this comfort zone is the 90% (PPD 10%) approach, i.e. 90% of all occupants must be comfortable with their internal conditions.
Fanger's comfort model based on PMV and PPD
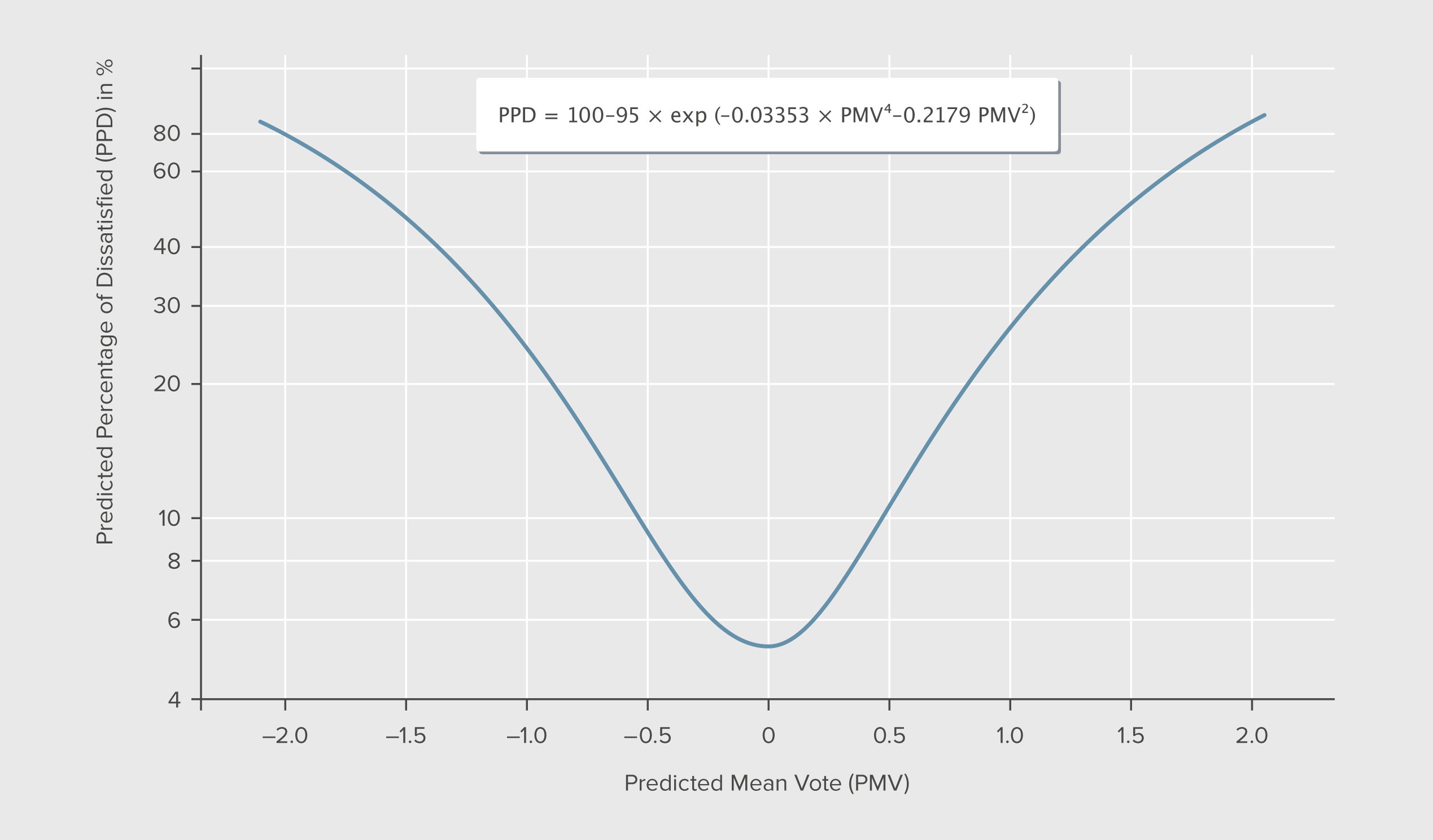
(Fanger, 1972)
ISO 7730 describes thermal comfort as: ‘that condition of mind which expresses satisfaction with the thermal environment’ (ISO 7730 and ASHRAE Standard 55). This standard uses the steady state heat balance of the human body to determine a range of comfort temperatures which occupants of buildings will find comfortable. The heat balance approach is defined exclusively by the internal condition of the building and the occupants’ state (clothing level and metabolic state). It is considered best applicable for actively conditioned indoor environments.
The adaptive approach is based on the natural tendency of people to adapt to changing conditions in their environment. This allows for enormous energy savings compared to the conventional approach of defining fixed indoor temperatures. The adaptive approach is in direct correlation with the outdoor conditions. The thermal comfort range for the adaptive approach is wider than that of the heat balance approach (de Dear and Brager, 1998; Nicol and Humphreys, 2002). Empirical equations are developed after typically carrying out thermal comfort surveys in occupants’ natural environment rather than climate-controlled chambers. This comfort zone also depends on the climate/ season or outdoor condition.
Adaptive comfort band

(de Dear and Brager, 1998)
During hot days, warmer interior temperatures are acceptable but during cold days, colder interior temperatures are acceptable. This will also reduce the thermal shock when moving between the indoor and outdoor environment. Standards such as ASHRAE-55 and EN15251 have provisions to determine thermal comfort using both heat balance approach and adaptive approach. Part of the psychological adaptation to warmer or cooler temperatures, is the ability to control the immediate environment, by opening or closing windows, activating or deactivating a fan. This is a crucial aspect that should not be underestimated. Adaptive approach is usually applied to determine thermal comfort conditions in naturally ventilated and mixed mode buildings.
Improvements
Thermal comfort in a space can be enhanced by passive measures such as increasing the air speed, by minimizing local discomfort factors such as radiant asymmetry, draft and thermal stratification. Each of these factors is influenced by the design architectural, interior design and air conditioning systems. Some of the measures are further described below.
In addition to providing good indoor air quality, ventilation of buildings has been a major cooling technique throughout the world. It is based on the fundamental heat-transfer mode of convection, where the air flowing next to a surface carries away heat, provided it is at a lower temperature than the surface. Increase in air speed is capable of offsetting a few degrees of temperature rise thus rising thermal comfort tolerance a few degrees higher than the normal.
When air passes over the human body, it increases the evaporation rate from the skin and enhances heat extraction. Even when the incoming air is at a higher temperature than the inside air, a positive effect on the thermal comfort conditions of the occupants can be achieved. For example, an increase of air velocity by 0.15 m/s compensates an increase of 1°C at a relative humidity of 75%. Air movement through buildings can be wind-induced or caused by differences in pressure due to a difference in temperature (stack effect). Hot air rises, which can then be extracted at the top, drawing fresh air into the building through an opening at the lower level. Empirical relation between dry bulb temperature, air velocity and relative humidity is given in the National Building code of India as follows:
Desirable wind speed (m/s) for thermal comfort conditions: Clause5.2.3.1, National Building Code of India, 2005
Dry bulb temperature (°C) |
Rh (%) |
Rh (%) |
Rh (%) |
Rh (%) |
Rh (%) |
Rh (%) |
Rh (%) |
|
30 |
40 |
50 |
60 |
70 |
80 |
90 |
28 |
* |
* |
* |
* |
* |
* |
* |
29 |
* |
* |
* |
* |
* |
0.06 |
0.19 |
30 |
* |
* |
* |
0.06 |
0.24 |
0.53 |
0.85 |
31 |
* |
0.06 |
0.24 |
0.53 |
1.04 |
1.47 |
2.10 |
32 |
0.20 |
0.46 |
0.94 |
1.59 |
2.26 |
3.04 |
** |
33 |
0.77 |
1.36 |
2.12 |
3.00 |
** |
** |
** |
34 |
1.85 |
2.72 |
** |
** |
** |
** |
** |
35 |
3.20 |
** |
** |
** |
** |
** |
** |
Source: (BIS, 2005); Rh = Relative humidity
However, air speed beyond a certain point, typically 1.6 m/s is not desirable as it causes unwanted turbulence. Moreover, thermal comfort is a complex phenomenon involving multiple factors and also being specific to geographical region and personal tolerances.
Radiant asymmetry is caused when there is considerable difference between the temperatures of different surfaces enclosing a space. Even though the space temperature is within the thermal comfort limits, this causes higher levels of radiant heat transfer on the occupant. For example, if somebody is seated close of the hot window or radiator, they experience discomfort on that areas of the body that are directly exposed to these high temperature surfaces. This can be minimised by achieving uniform surface temperatures for al the surfaces in a space.
Draft is experienced when there is unwanted air movement directed over specific area of the body or the entire body. This could be a cold or hot draft or simply air speeds beyond acceptable limits. Draft can also result when occupants are placed in close proximity to air conditioning diffusers in the space. Draft can be minimised by making the building envelope airtight and by designing the diffusers, regulating air speed from diffusers and their placement so as to avoid draft.
Thermal stratification is experienced when the air in the space is not well mixed and temperature difference can be experienced at the head level compared to the ankle level. Thermal stratification is particular experienced in spaced with active slab heating or cooling where floor slab or the roof/ceiling is actively heated or cooled. Care should be taken that the stratification is more prominent beyond the head level of the occupants and that a fairly uniform temperature is maintained within the occupied zone, i.e., from head to toe.
Authors
- Johanna Knaak
- Sriraj Gokarakonda
- Christopher Moore
References
- BIS, (2005). National building code of India, 2005. 2nd ed. New Delhi: Bureau of Indian Standards, pp.8-1-36.
- de Dear, R. and Brager, G. (1998). Developing an adaptive model of thermal comfort and preference. ASHRAE Transactions, 104(1).
- Fanger, P. (1972). Thermal comfort: analysis and applications in environmental engineering. New York: McGraw-Hill.
- Griffiths, I. (1988). Field-Based Research on Thermal Comfort in Passive Solar Buildings. In: T. Steemers, ed., Solar Energy Applications to Buildings and Solar Radiation Data: Proceedings of the EC Contrac-tors’ Meeting held in Brussels, Belgium, 1 and 2 October 1987, 1st ed. Dordrecht: Kluwer Academic Pub-lishers., pp.110-114.
- Harvey, L. (2010). Energy and the new reality. London: Earthscan.
- Lin, Z. and Deng, S. (2004). A study on the characteristics of nighttime bedroom cooling load in tropics and subtropics. Building and Environment, 39(9), pp.1101-1114.
- Nicol, J. and Humphreys, M. (2002). Adaptive thermal comfort and sustainable thermal standards for buildings. Energy and Buildings, [online] 34(6). Available at: http://www.sciencedirect.com/science/article/pii/S0378778802000063 [Accessed 31 Aug. 2016].
- Olgyay, V. (1963). Design with climate. [S.l.]: Princeton Univ Press.
- Szokolay, S. (2008). Introduction to architectural science. Oxford [etc.]: Architectural Press, p.17.